Unexpected warming induced by foehn winds in the lee of the Smoky Mountains
by
David M. Gaffin
ABSTRACT
On 2 January 1999, a southeasterly foehn wind produced a narrow band of temperatures up to 100C (180F) warmer than the surrounding area in the central Great Tennessee Valley on the northwest side of the Smoky Mountains. This particular event was interesting compared to other documented foehn wind events in that the dewpoint temperature rose substantially along with the actual temperature, resulting in little change in the observed surface relative humidity. A relatively warm and nearly saturated 850 hPa airmass around the highest ridges of the Smoky Mountains was found to be the source of the adiabatically compressed air observed on the northwest side of the Smoky Mountains.
- The observed foehn winds across the central Great Tennessee Valley were not unusually strong during the afternoon, with surface wind gusts reaching 13 m s-1 (26 kt). However, mountain waves may have been associated with the foehn winds on 2 January 1999, since a strong surface pressure gradient was in place across the mountain range, winds in excess of 15 m s-1 (30 kt) were observed in a stable layer at 850 hPa blowing nearly perpendicular to the highest Smoky Mountain ridges, and favorable vertical wind and terrain profiles were present. Any mountain wave activity likely remained close to the mountains away from any of the observation stations in the Great Tennessee Valley. Also, high relative humidity levels (>70%) throughout much of the boundary layer near the Great Tennessee Valley may have reduced the strength of the foehn winds.
1. Introduction
- Foehn winds are commonly observed in the lee of large mountain ranges and are typically associated with dramatic temperature increases and relative humidity decreases due to adiabatic compression of the descending wind. Foehn winds have been frequently documented near the mountains of the western United States, and include the Chinook winds near the Rocky Mountains (Oard 1993), the Santa Ana winds near the mountains of southern California (Burroughs 1987; Lessard 1988), and the Sundowner winds near the Santa Ynez Mountains (Blier 1998; Ryan and Burch 1992). Foehn winds observed across the western United States can be quite strong and produce an abrupt increase in temperature and decrease in relative humidity as they descend (Blier 1998; Oard 1993; Nkemdirim 1986). It has been theorized that in some foehn events, upslope winds on the windward side of the mountains induce the release of latent heat due to condensation and precipitation, contributing to the relatively warm and dry winds observed on the leeward side. Mountain wave activity is thought to occur with most foehn wind events in the western United States (Blier 1998; Oard 1993), contributing to the strong gusty winds observed during these events.
- Large temperature rises due to the adiabatic compression associated with foehn winds are unusual near the Appalachian Mountains, since the Appalachians have less relief and more gentle slopes in general than the Rockies and the Alps and, thus, a smaller potential for adiabatic warming. However, the Smoky Mountains are some of the highest mountains in the Appalachian range, with peaks rising to nearly 1500 m (5000 ft) above the Great Tennessee Valley (Fig. 1). As will be seen in this case study, significant temperature rises can be produced downwind of the Smoky Mountains by foehn winds.
2. Meteorological Conditions on 2 January 1999
- On 2 January 1999, an isolated mesoscale region of relatively warm temperatures was reported across the central Great Tennessee Valley. Knoxville McGhee-Tyson Airport (Fig. 2) reported a high temperature of 150C (590F), the Knoxville Downtown Island Airport reported 160C (610F), and the Gatlinburg-Pigeon Forge Airport reported 160C (600F). Surrounding sites in east Tennessee reported highs only in the 5 to 100C range (70C (450F) at Chattanooga, 90C (480F) at Oak Ridge, and 80C (460F) at Tri-Cities), with highs around 00C along the eastern slopes of the southern Appalachian Mountains (for example, -10C (310F) at Asheville). Other sites in the central Great Tennessee Valley recorded high temperatures just above 100C (Athens 120C (540F) and Morristown 110C (520F)), while sites farther northeast, such as Greeneville and Elizabethton, reported highs of only 70C (450F) and 60C (420F), respectively. The significantly warmer temperatures around 160C (600F), approximately 100C (180F) above the surrounding area temperatures, occurred in a narrow band to the northwest of the Smoky Mountains.
- Surface hand analyses on 2 January 1999 (Fig. 3) revealed a cold air damming situation east of the southern Appalachian Mountains associated with a stagnant high pressure wedge over the Carolinas and northern Georgia. Temperatures remained in the negative single digits 0C (mid 20s to lower 30s0F) most of the afternoon across the Carolinas on the eastern side of the southern Appalachian Mountains. On the western side of the southern Appalachian Mountains, warmer air between 5 and 100C (40s0F) was in place across the Great Tennessee Valley in advance of an eastward moving low pressure system over the mid-Mississippi River Valley. A strong pressure gradient across the southern Appalachian region helped to produce southeast winds across the Great Tennessee Valley that gusted to 13 m s-1 (26 kt) near the foothills of the Smoky Mountains. These southeast winds helped delay the progression of the surface warm front over middle Tennessee during the afternoon hours, preventing it from entering the Great Tennessee Valley until the evening hours.
- Morning soundings (Fig. 4) from around the southern Appalachian region at 1200 UTC (0700 LST) revealed a stable airmass with a deep temperature inversion extending up to 700 hPa. This inversion extended above the highest ridges of the Smoky Mountains, which rise approximately 330 m (1000 ft) above the 850 hPa level. A sub-freezing and dry airmass between 900 and 700 hPa was located east and northeast of the southern Appalachian Mountains (Figs. 4c and 4d), while a relatively warm and nearly saturated airmass between 850 and 700 hPa was located to the west and south of the southern Appalachian Mountains (Figs. 4a and 4b). The 1200 UTC (0700 LST) 850 hPa analysis (Fig. 5a) revealed a south-southeasterly wind flow bringing nearly saturated air with temperatures around 70C (450F) across the southern Appalachian region. The 1200 UTC (0700 LST) 500 hPa analysis (Fig. 5b) revealed a closed low centered over South Dakota creating a southwest flow across the southern Appalachian region.
3. Discussion
- i) Cause of the observed warming
- The Gatlinburg-Pigeon Forge Airport and Knoxville McGhee-Tyson Airport observing sites near the foothills of the Smoky Mountains (Table 1) began to report southeast winds and a resultant rise in temperatures around 1800 UTC (1300 LST) as the surface pressure gradient strengthened. Knoxville Downtown Island Airport, further northwest in the central Great Tennessee Valley, began to report southeast winds and a noticeable resultant temperature rise by 2100 UTC (1600 LST). These winds shifted to the west and temperatures dropped around 2300 UTC (1800 LST) at Knoxville's McGhee-Tyson and Downtown Island airports as outflow from rain ahead of the warm front over middle Tennessee ended the adiabatic warming from the southeast winds. The fact that the Knoxville winds were from the southeast until 2300 UTC (1800 LST) while temperatures further south and west at Chattanooga and Oak Ridge remained in the upper single digits 0C (mid to upper 40s0F) indicates that the surface warm front located to the south and west did not influence the observed warming in the central Great Tennessee Valley during the afternoon.
- The National Weather Service operational Eta model, (32 km resolution and 45 vertical levels) initialized at 1200 UTC (0700 LST) on 2 January 1999, was examined to determine the amount of warming forecasted to occur at 850 hPa around 1800 UTC (1300 LST), when the maximum warming observed across the central Tennessee Valley began. The 6-hour Eta model forecast of 850 hPa winds and temperatures (Fig. 6) valid at 1800 UTC (1300 LST) indicated strong southerly winds in excess of 30 m s-1 (60 kt) and a relatively warm airmass with temperatures around 60C (430F) across the Smoky Mountains. The Eta model's 850 hPa winds at 1800 UTC (1300 LST) across middle Tennessee were likely around 5 m s-1 (10 kt) too strong when its initialization is compared to the 1200 UTC (0700 LST) upper-air analysis across the southern United States. However, the Eta model's 850 hPa temperature initialization appeared to be similar to 1200 UTC (0700 LST) observations, which indicated that the 1800 UTC (1300 LST) forecast was close to reality.
- Following the dry adiabatic lapse rate of 9.80C km-1 (5.40F 1000 ft-1), an air parcel at 6 to 70C (43 to 450F) forced down the average descent in the Smoky Mountains of 1000 m (3300 ft) should theoretically warm 9.80C (17.80F) adiabatically. This theoretical result indicates that temperatures between 15.80C (600F) and 16.80C (620F) would have been observed across the central Tennessee Valley, which compares well to the observed narrow band of warmer temperatures around 160C (600F). The higher Smoky Mountains created a more effective dam of the colder surface air over the Carolinas, compared to the rest of the southern Appalachian Mountains. This explains why the rest of the Great Tennessee Valley did not also observe a noticeable warming of temperatures with southeast winds.
- The temperature rises were also accompanied by substantial dewpoint temperature rises to between 70C and 90C (450F and 480F) (Table 1) across the central Great Tennessee Valley. The nearly saturated 850 hPa airmass, with dewpoints around 60C (430F), was most likely the source region for the narrow band of warm temperatures observed downwind of the Smoky Mountains. In contrast, on the eastern side of the southern Appalachian Mountains surface weather stations reported a northeast wind with dewpoints in the -6 to -10C (21 to 300F) range throughout the afternoon, indicating that this airmass was not the source of the observed warming in the central Great Tennessee Valley. With the mixing ratio of the 850 hPa airmass conserved in an adiabatic descent, dewpoint temperatures would have theoretically risen approximately 20C during a 1000 m descent. This matches well with the observed dewpoint temperature increases to between 70C and 90C (450F and 480F).
- Visible satellite images from 2 January 1999 (Fig. 7) indicated that solar heating did not have a major impact on the observed temperature rises during the afternoon in the central Great Tennessee Valley. The warming to around 100C (510F) observed across southeast Kentucky during the afternoon hours (Fig. 3) was likely the result of solar heating caused by the substantial clearing of cloud cover in this area. Although some breaks in the cloud cover occurred over the central Great Tennessee Valley around 1902 UTC (1402 LST), surface observations across the central Great Tennessee Valley reported broken to overcast high clouds throughout the afternoon. The descending motion created by the southeast winds only eroded the low to mid level clouds (from around 850 hPa to the surface), while most of the high clouds (above 850 hPa) remained throughout the afternoon, limiting the influence of solar heating on the observed warming in the central Great Tennessee Valley. The satellite images at 1702 UTC (1202 LST) and 1902 UTC (1402 LST) also indicated some signs of banding in a mountain-parallel direction, which would support the possibility of mountain wave activity across the central Great Tennessee Valley.
-
- ii) Possibility of mountain waves
- Most strong foehn wind events in the western United States are associated with mountain wave activity (Blier 1998; Oard 1993), which contributes to the strong gusty surface winds normally observed during these events. Previous research has found certain conditions which increase the likelihood of mountain waves, including a cross-barrier wind flow that is roughly 300 of perpendicular to the ridge line, cross-barrier wind speed exceeding a terrain-dependant value of 7 to 15 m s-1 (14 to 30 kt), and an inversion extending above the mountain ridge with weaker stability at higher levels in the upstream environment (Queney et al. 1960). Klemp and Lilly (1975) also confirmed that these conditions were important in the generation of wave induced downslope wind events. Other factors which have been found to enhance the occurrence of mountain waves include a vertical wind profile in which the cross-mountain wind component increases with height (Scorer 1949; Colson 1954) and the existence of a mountain barrier with a gentle windward slope and a steep leeward slope (Queney et al. 1960; Lilly and Klemp 1979).
- On 2 January 1999, mountain wave activity may have been associated with the foehn winds observed across the central Tennessee Valley as many favorable conditions for the formation of mountain waves were in place. A strong surface pressure gradient was present across the mountain range along with a strong inversion extending above the highest mountain ridges and capped by a large conditionally unstable layer. Also, a strong southerly 850 hPa wind exceeding 15 m s-1 (30 kt) was nearly perpendicular to the highest mountain ridges of the Smoky Mountains (which are generally oriented along a west-to-east axis). The morning soundings on 2 January 1999 revealed a vertical wind profile in which the cross-mountain wind component increased with height, while the terrain profile of the Smoky Mountains enhanced the possibility of mountain waves with relatively gentle rising slopes to the southeast and a steep descent on the northwest side into the Great Tennessee Valley.
- However, surface wind gusts across the central Great Tennessee Valley peaked at approximately 13 m s-1 (26 kt) throughout the afternoon on 2 January 1999. The absence of stronger wind gusts indicated that any mountain wave activity likely remained close to the mountains and did not traverse any of the observation stations in the valley. Also, Durran and Klemp (1983) found that high relative humidity in the lower troposphere appeared to reduce the strength of downslope winds. This could also help explain the strength of the wind gusts observed on 2 January 1999, since the Great Tennessee Valley likely possessed high relative humidity levels (>70%, inferred from the Atlanta and Nashville soundings) throughout much of the boundary layer and especially around the 850 hPa level.
4. Conclusions
- The narrow band of warm temperatures, up to 100C (180F) above the surrounding area temperatures, observed on 2 January 1999 across the central Great Tennessee Valley was the result of southeasterly foehn winds in the lee of the Smoky Mountains. The peak period of warming was during the afternoon hours, when the surface pressure gradient strengthened over the Great Tennessee Valley as a low pressure system over the mid-Mississippi Valley approached the high pressure cold air damming situation over the Carolinas. This event was interesting compared to other documented foehn winds in that the dewpoint temperature rose substantially along with the actual temperature, resulting in little change in the observed surface relative humidity. The relatively warm and nearly saturated 850 hPa airmass was the source region of the adiabatically compressed air observed in the lee of the Smoky Mountains. This nearly saturated 850 hPa airmass, with temperatures between 60C (430F) and 70C (450F), contributed to the magnitude of the warming and the increase in moisture observed across the central Great Tennessee Valley. The significant warming was limited to the central Great Tennessee Valley since the higher Smoky Mountains dammed the colder surface air over the Carolinas more effectively compared to the rest of the southern Appalachian Mountains.
- Mountain wave activity, typically observed with other documented foehn winds, may have occurred during the afternoon of 2 January 1999. The presence of a strong surface pressure gradient across the Smoky Mountains, an inversion extending above the mountain ridges and capped by a conditionally unstable layer, a strong 850 hPa wind in excess of 15 m s-1 (30 kt) nearly perpendicular to the highest mountain ridges, and a favorable vertical wind and terrain profile enhanced the possibility of mountain waves. Visible satellite images from the afternoon of 2 January 1999 also revealed some mountain-parallel cloud banding which further supported the possibility of mountain waves. The fact that the observed surface winds gusts reached only 13 m s-1 (26 kt) across the central Great Tennessee Valley was likely the result of any mountain wave activity remaining close to the mountains away from the observing stations in the valley. Also, the surface winds could have been reduced by high relative humidity levels (>70%) observed throughout much of the boundary layer near the Great Tennessee Valley.
- The magnitude of this event was unexpected and had a significant impact on the temperature forecast for the central Great Tennessee Valley. Local forecasters will have a formidable task in predicting the onset and magnitude of these foehn wind events in the lee of the Smoky Mountains. In order to most effectively anticipate a foehn wind event in the southern Appalachian region, local forecasters should examine synoptic conditions for the presence of a relatively warm flow of air at 850 hPa, a strong surface pressure gradient across the mountain range, winds in excess of 15 m s-1 (30 kt) around 850 hPa blowing nearly perpendicular to the mountain ridges, a strong inversion extending above the mountain ridges and capped by a layer of weaker stability, and a vertical wind profile in which the cross-mountain wind component increases with height. With knowledge of the synoptic conditions which enhance the possibility of foehn wind events, operational forecasters will have a reasonable chance of anticipating the onset of such events.
Acknowledgments
- The author would like to thank Stephen Parker (Science and Operations Officer at WFO Morristown) for his assistance in obtaining the surface and satellite data, and Dolores Kiessling (COMET-UCAR) for providing the Eta model data. Also, thanks to Stephen Parker, Dr. George Young (The Pennsylvania State University), and two anonymous reviewers for their helpful and constructive reviews of this manuscript.
References
- Blier, W., 1998: The Sundowner winds of Santa Barbara, California. Wea. Forecasting, 13, 702-716.
- Burroughs, L. D., 1987: Development of forecast guidance for Santa Ana conditions. Natl. Wea. Dig., 12, 4-11.
- Colson, D., 1954: Meteorological problems in forecasting mountain waves. Bull. Amer. Meteor. Soc., 35, 363-371.
- Durran, D. R., and J. B. Klemp, 1983: A compressible model for the simulation of moist mountain waves. Mon. Wea. Rev., 111, 2341-2361.
- Klemp, J. B., and D. K. Lilly, 1975: The dynamics of wave-induced downslope winds. J. Atmos. Sci., 32, 320-339.
- Lessard, A. G., 1988: The Santa Ana winds of southern California. Weatherwise, 41, 100-104.
- Lilly, D. K., and J. B. Klemp, 1979: The effects of terrain shape on nonlinear hydrostatic mountain waves. J. Fluid Mech., 95, 241-261.
- Nkemdirim, L. C., 1986: Chinooks in southern Alberta: Some distinguishing nocturnal features. J. Climatol., 6, 593-603.
- Oard, M. J., 1993: A method for predicting chinook winds east of the Montana Rockies. Wea. Forecasting, 8, 166-180.
- Queney, P., G. Corby, N. Gerbier, H. Koschmieder, and J. Zierep, 1960: The airflow over mountains. WMO Tech. Note 34, 135 pp. [Available from World Meteorological Organization, P.O. Box 2300, CH-1211 Geneva 2, Switzerland.]
- Ryan, G., and L. E. Burch, 1992: An analysis of Sundowner winds: A California downslope wind event. Preprints, Sixth Conf. on Mountain Meteorology, Portland, OR, Amer. Meteor. Soc., 64-67.
- Scorer, R., 1949: Theory of waves in the lee of mountains. Quart. J. Roy. Meteor. Soc., 75, 41-56.
Table 1. Surface observations on 2 January 1999.
Gatlinburg-Pigeon Forge Airport |
Time (UTC) |
Cloud heights (km) and sky coverage |
Temp/Dewpt (oC) |
Wind Direction |
Wind Speed (m s-1, G=gusts) |
Pressure (hPa) |
1400 |
3.7 CLR |
3 / -2 |
|
Calm |
1015.6 |
1500 |
1.5 SCT |
7 / 0 |
|
Calm |
1014.9 |
1600 |
1.5 SCT, 2.6 SCT |
9 / 2 |
090o |
1.5 |
1014.2 |
1700 |
1.5 BKN |
12 / 4 |
050o |
1.5 |
1012.2 |
1800 |
1.5 OVC |
14 / 7 |
150o |
3.0 |
1009.8 |
1900 |
1.4 SCT, 2.7 BKN |
15 / 7 |
120o |
5.5 G 9.0 |
1007.8 |
2000 |
1.5 BKN |
16 / 7 |
120o |
6.0 G 9.5 |
1006.1 |
2100 |
1.5 BKN, 2.3 BKN, 2.4 BKN |
15 / 7 |
110o |
4.5 G 7.5 |
1005.4 |
2200 |
1.1 SCT, 1.5 SCT, 2.7 BKN |
13 / 7 |
100o |
2.5 G 7.0 |
1004.7 |
2300 |
1.5 SCT, 2.6 BKN |
14 / 7 |
140o |
4.5 G 7.0 |
1003.7 |
Knoxville McGhee Tyson Airport |
Time (UTC) |
Cloud heights (km) and sky coverage |
Temp/Dewpt (oC) |
Wind Direction |
Wind Speed (m s-1, G=gusts) |
Pressure (hPa) |
1400 |
1.1 FEW, 2.4 SCT |
2 / -2 |
020o |
2.0 |
1015.1 |
1500 |
1.8 SCT, 2.1 BKN |
4 / -3 |
340o |
3.5 |
1014.6 |
1600 |
1.5 FEW, 2.3 SCT, 2.9 OVC |
6 / -2 |
340o |
3.0 |
1013.8 |
1700 |
1.5 BKN, 2.0 OVC |
10 / 2 |
Variable |
2.5 |
1011.2 |
1800 |
1.3 SCT, 1.8 BKN, 2.9 OVC |
14 / 7 |
140o |
5.0 G 12.0 |
1008.4 |
1900 |
1.3 BKN, 1.8 BKN |
14 / 7 |
160o |
8.5 G 11.5 |
1006.4 |
2000 |
1.4 SCT, 2.4 SCT, 2.7 BKN |
14 / 7 |
160o |
6.0 G 12.5 |
1004.4 |
2100 |
2.4 BKN, 2.9 BKN |
13 / 7 |
150o |
8.5 G 11.5 |
1003.4 |
2200 |
1.2 SCT, 1.7 SCT |
13 / 8 |
150o |
4.5 |
1003.1 |
2300 |
0.9 SCT, 1.1 BKN, 2.3 OVC |
9 / 8 |
270o |
10.0 G 12.5 |
1004.7 |
Knoxville Downtown Island Airport |
Time (UTC) |
Cloud heights (km) and sky coverage |
Temp/Dewpt (oC) |
Wind Direction |
Wind Speed (m s-1, G=gusts) |
Pressure (hPa) |
1400 |
2.1 BKN |
5 / -4 |
080o |
3.0 |
1015.6 |
1500 |
2.0 BKN, 2.3 OVC |
6 / -4 |
080o |
2.5 |
1015.2 |
1600 |
2.3 SCT, 2.7 OVC |
8 / -2 |
070o |
2.0 |
1014.2 |
1700 |
2.1 BKN, 2.7 OVC |
9 / 1 |
070o |
2.5 |
1011.9 |
1800 |
1.5 BKN, 2.7 OVC |
11 / 3 |
070o |
3.0 |
1008.8 |
1900 |
1.5 OVC |
13 / 3 |
350o |
1.5 |
1007.1 |
2000 |
1.5 SCT, 2.0 BKN, 2.1 BKN |
13 / 5 |
250o |
2.0 |
1005.4 |
2100 |
1.5 SCT, 2.3 BKN, 2.6 OVC |
16 / 8 |
130o |
4.5 |
1004.1 |
2200 |
1.5 OVC |
16 / 8 |
140o |
2.5 |
1004.1 |
2300 |
0.2 SCT, 1.2 BKN, 2.4 OVC |
11 / 8 |
290o |
6.5 G 12.0 |
1005.8 |
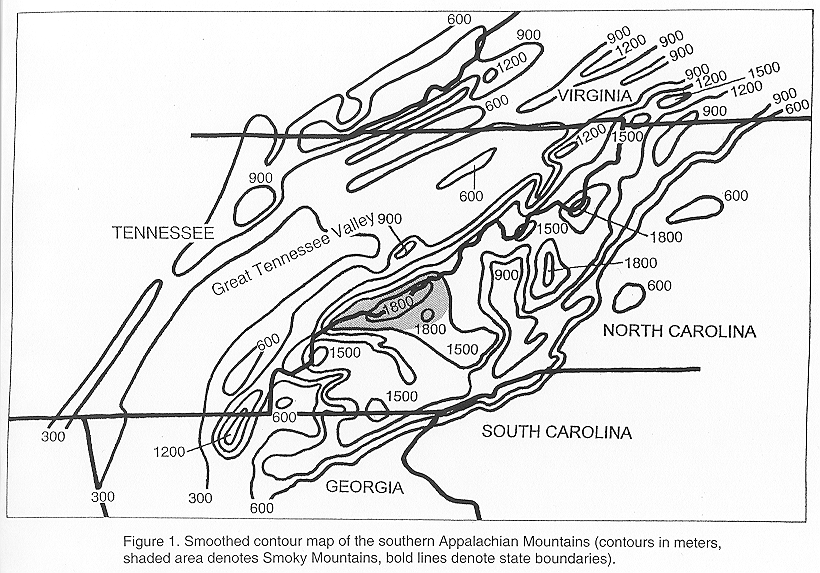
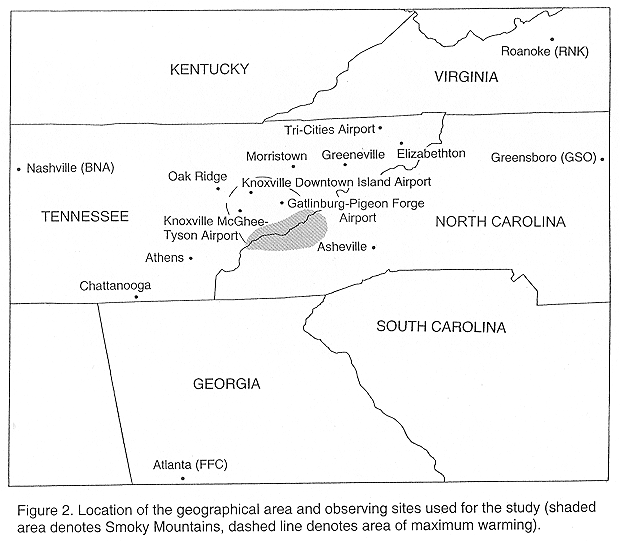
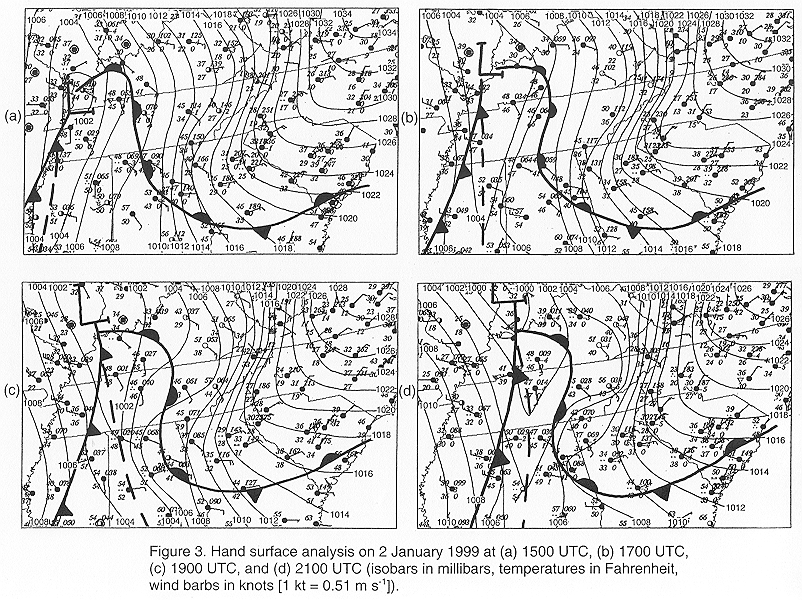
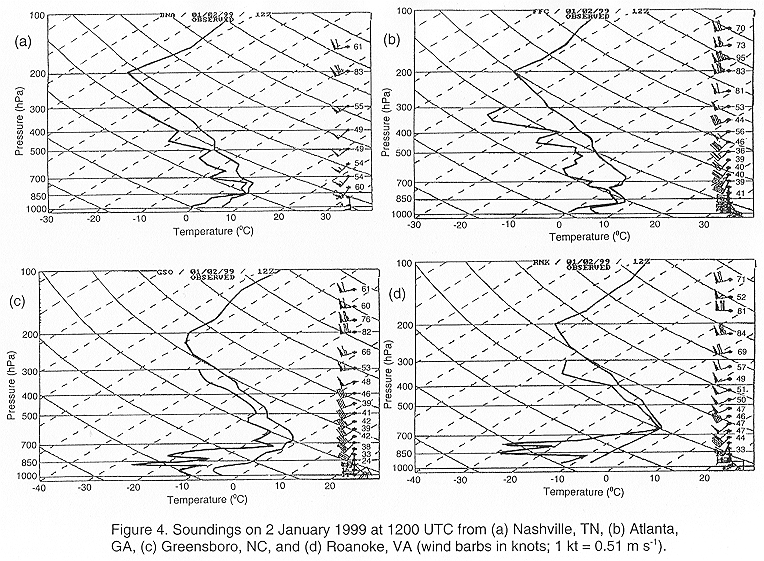
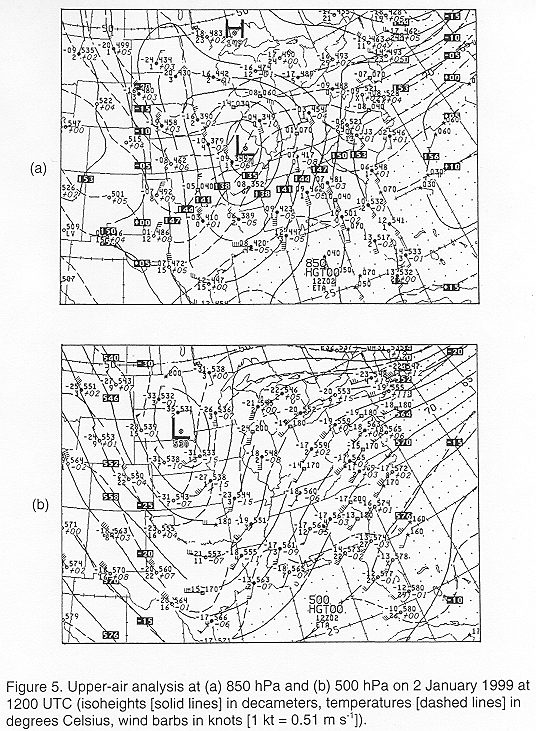
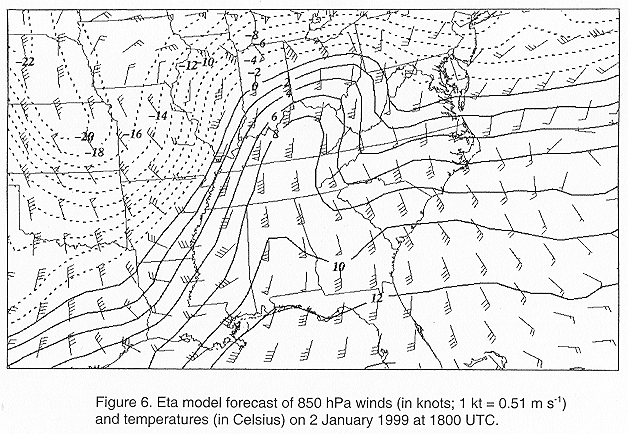
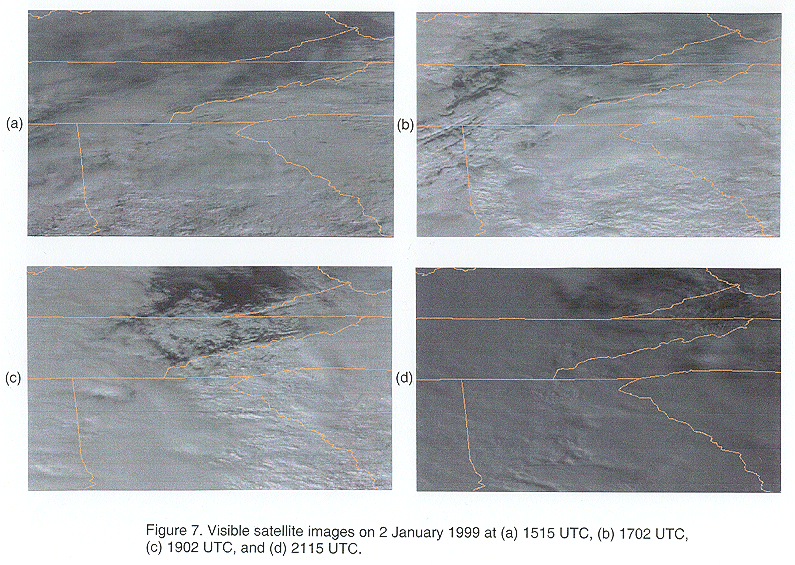